Overview of This Section
The previous two segments dealt with free radical and anionic vinyl polymerizations in which the active chain end was either a free radical or an anion. This segment will examine the use of cations, and specifically carbocations, as propagating chain ends.
Let's dive right into the differences in the initiation, propagation and termination reactions of this type. In fact, this kind of polymerization is the only way to make certain commercial polymers. Similar to the other types, though, reaction of the cationic chain end with a vinyl double bond forms a new single bond to one of the carbons, leaving the other with shy a pair of electrons and that makes the active chain end even more reactive. And as before, this reaction goes on, over and over and over to make high polymer.
Polymers made by cationic polymerization include polystyrene, polyisobutylene, polybutadiene and several types of vinyl ethers. So let's get to it...
Cationic Chain-growth Details
You're probably wondering how the "match" in this case is different. The types of cationic initiators fall into two main categories: protonic acids and Lewis acids. Each type works best for a specific family of monomers although often either can be used. Let's look more closely at the various initiators available.
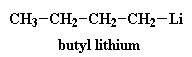
Now a little bit of the butyl lithium in solution will fall apart or dissociate. Not a lot, but some. It falls apart to form a positive lithium cation and a negative butyl anion. We call an anion like this where the negative charge is on a carbon atom a carbanion.

When this very reactive carbanion comes near a vinyl group, the non-bonded (ionic) pair of electrons from the butyl anion will be donated to attack one of the double bond carbon atoms of the monomer. Now this carbon atom already has eight electrons in its outer shell which it shares with the atoms to which it is bonded, so one pair of these electrons, specifically the pair in the carbon-carbon double bond, will leave that bond and settle on the other carbon atom of the carbon-carbon double bond. This forms a new carbanion, with the negative charge resting on that carbon. The process in which the butyl lithium dissociates and the butyl anion reacts with a monomer molecule is called initiation.
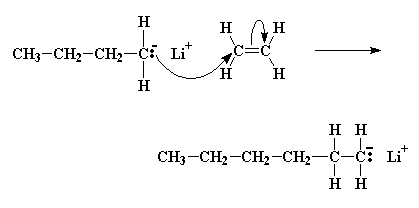
Click here to see a movie of the initiation reactions.
The carbanion now reacts with another monomer molecule in just the same manner as the initiator reacted with the first monomer molecule; another carbanion is generated. This keeps happening, and each time another monomer is added to the growing chain, a new anion is generated allowing another monomer to be added. In this way the polymer chain grows. This adding of monomer after monomer is called propagation.
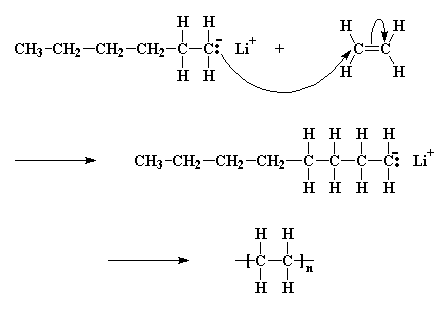
Click here to see a movie of the propagation reactions.
The Chain That Wouldn't Die
Now you may get this nagging feeling that this can't go on forever, that something has to put a stop to this convenient process. Funny thing, though: it doesn't stop! In many cases, the only thing that stops monomers from adding to the growing chain, is that eventually there are no more monomer molecules in the reactor left to add! And even then, if someone came along some time later and dumped more monomer in, the new monomers would add to the chain and the chain would grow some more! Some chains of polystyrene have been known to stay active like this for years. In order to finally stop them and quench the active chain ends, something like water is added to react with the carbanions. In general, polymerization systems like this are called living anionic polymerizations. This "livingness" allows us to do some interesting tricks
Modular Chemistry
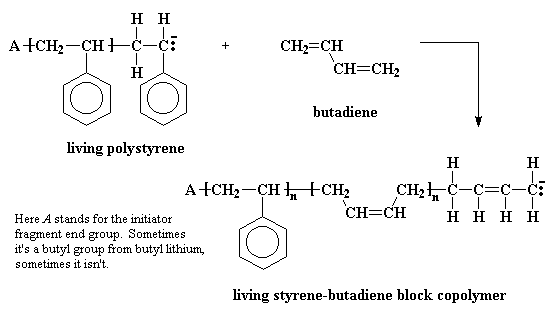
Some time ago someone was pondering this beaker full of polymer which would stay active for years; and how if one added additional monomer, this new monomer would add to the living polymer chains, and came up with an idea. Instead of adding the same monomer to the solution filled with living polymer, why not add a different monomer? The result was a polymer whose chains consisted of a long stretch of one type of polymer, and a second long stretch of another polymer. Polymers like this are called block copolymers. For example, a solution of living polystyrene chains will react with butadiene to give a styrene-butadiene block copolymer.
A few more tricks will give us a styrene-butadiene-styrene triblock copolymer. Don't you wish you knew how we do this? To find out click here.
The first thing we have to do is make a chain of living polystyrene. This is done by polymerizing the monomer styrene with an anionic initiator like butyl lithium.
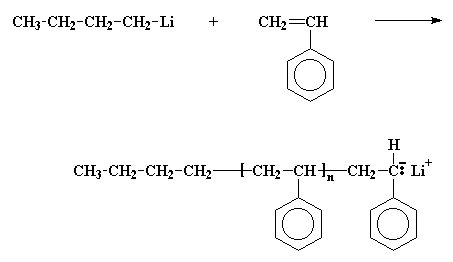
Remember now, this polystyrene chain is living, so if we add a second monomer to it, it'll add to the polymer. So we'll add some of the monomer butadiene.
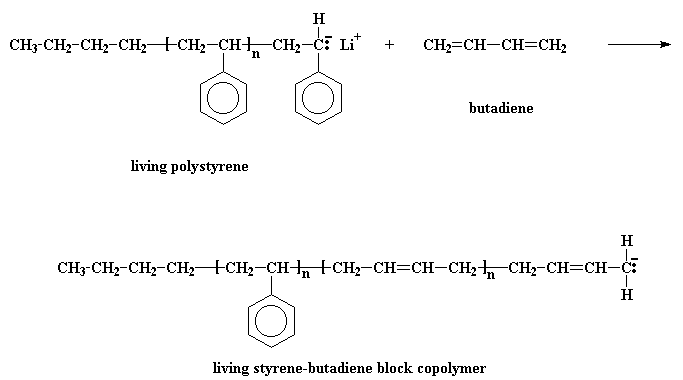
That gives us a living styrene-butadiene block copolymer.
The next step is obvious: just add more styrene monomer, and get a styrene-butadiene-styrene triblock copolymer. Easy as pie. Funny thing, though. Although butadiene monomer will add to the anion at the end of a polystyrene chain, styrene monomer won't add easily to the anion at the end of a living polybutadiene chain. This is most inconvenient. To get around this, we do a little trick: we're going to react it with a compound called dichlorodimethylsilane.
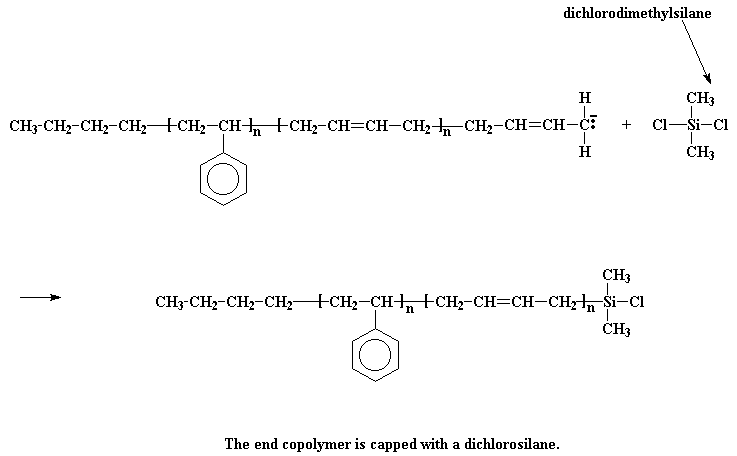
The anionic living chain kicks off a chlorine atom from the silane, and we get a chlorosilane end-capped polymer. So what good is that? Now our polymer is no longer living! It's good because we can do something with this chlorosilane end-capped polymer. You see, if we take living polystyrene homopolymer, it will react with the chlorosilane end-capped polymer, just like the styrene-butadiene copolymer reacted with the dichlorodimethylsilane.
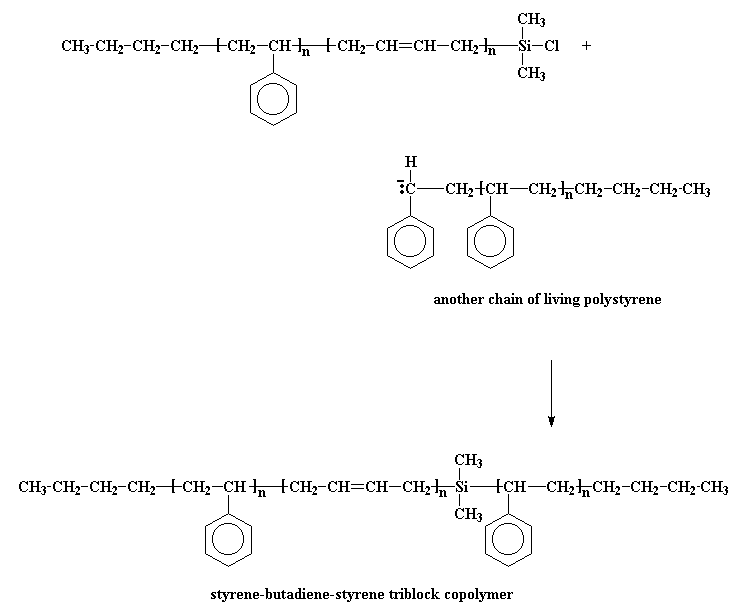
This gives us the triblock copolymer we wanted. Right nifty, if you ask me.
Oh, so you want to know why we didn't just add more of the first monomer, styrene to the living diblock chain instead of the silane terminating group? Great questions! And the answer is: that works, too. And that method is used with other monomers as well.
You could add a third (different) monomer to the living diblock polymer before adding the silane, and that would have given you a triblock with each block being different. People (scientists, I'm sure you guessed) have done this ad nauseum and made polymers with five, seven and even more different or the same blocks in all kinds of combinations and chain segment lengths.
This kind of anionic polymerization gives you tremendous capability to make multi-block copolymers. The only stipulation should be obvious: all the monomers must be capable of anionic polymerization. Can you think of any way around that requirement? Scientists have, but we'll leave you wondering for now...
the fun-filled, fact-filled and just plain fascinating polyethylene page.
The term "chain-growth" refers to how polymer chains are actually formed. With step-growth, all polymers grow slowly more or less at the same time and rate. With chain-growth, polymers are made one chain at a time. That is, whatever's in your reaction vessel is either monomer or high molecular weight polymer. There aren't any oligomers (unless you do things wrong like Neo did) and you could stop the reaction at any time, precipitate the polymeric component and filter it from the left over monomer, and it should be perfectly usable.
In actual fact, it's almost impossible to use up all the monomer in this type of polymerization. Why? Simple reason is that as conversion gets high, there's not enough monomers left to find the active chain end to add to it. The rate of polymerization grinds to a halt (so much for whetting your appetite) and you might as well just end it so you can get your polymer out.
Another very important aspect of this type of polymerization is that things happen fast. Chains literally "zip up" as fast as monomer can find an active chain end. Oh, but there can't be very many of those because they react with each other, at least for radical polymerizations.
Let me say that a different way: you only can start so many chains at any given time if you want high molecular weight. Start too many and the ends find each other, couple and they can't react any more. Problem is, the more active ends there are, the faster is conversion, which is desirable from an industrial perspective. But if the reaction goes too fast, things get out of hand: exotherms that vaporize monomer and blow the lid off your reaction vessel.
Confused yet? It's not really all that complicated. Why not make a list or a table with the characteristics and consequences of each type of polymerization. That will help you see key differences and why you do each the way you do so you get usable polymer without blowing anything up. I'd do it for you, but the exercise is good for you (heard that before, have you?). On to cationic.
Quiz Time Again!
Right now, though, it would help you a lot to know what you DO know and more to the point, what you DON’T know. You should be starting to like taking quizzes, since they’re actually fun! And they really help you understand where you are in the learning process. So let’s take one, ok?
This quiz is about free radical polymerization. Don't be afraid to guess- your subconscious will often times pop up the right answer even if you're not sure of it: classification evaluation quiz.
And when you're ready to move on, you can begin exploring the details of cationic vinyl polymerizations which are very different from their radical and anionic cousins. These are still chain-growth and addition polymerizations, so checking out the differences among these three is helpful in understanding how to make specific polymers made only with one or two of the methods.
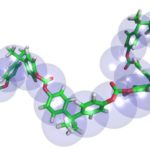