Turns out there are two main types of polyesters based on components in the repeat units that contribute to backbone flexibility or stiffness (see the figure below). And actually, there's a third general type, unsaturated esters such as acrylates that become ester-containing vinyl polymers; we'll discuss those later.
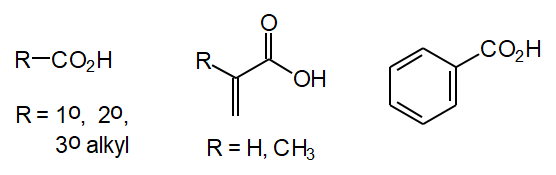
Aliphatic polyesters are flexible, low Tg and Tm materials with reasonable physical properties. The table below lists some common aliphatics polyesters as of a few years ago. Note that some have changed hands or otherwise been relocated somewhere in the polymer universe.
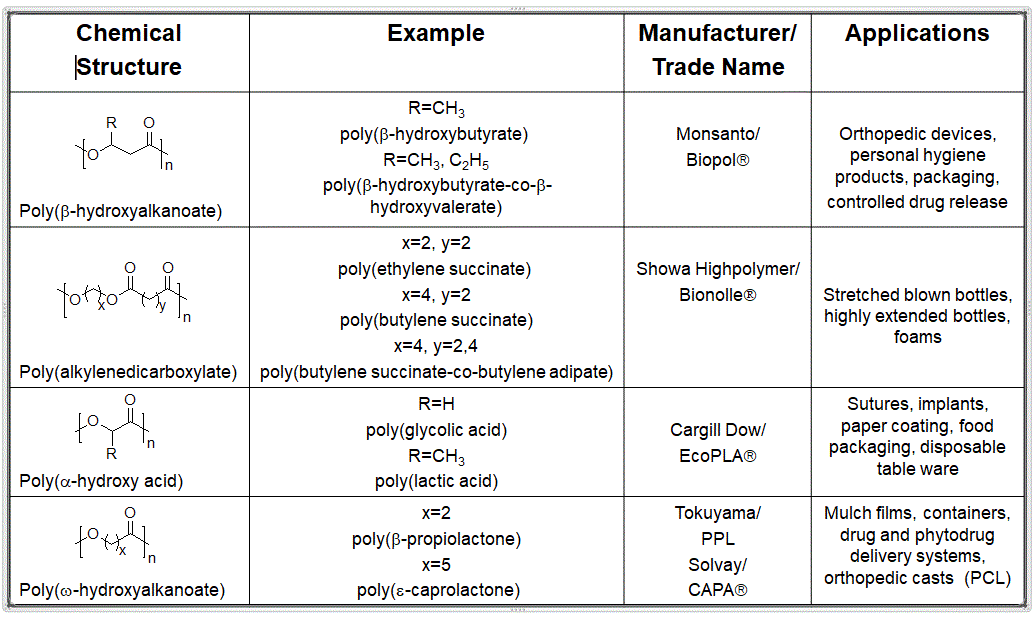
Aromatic polyesters, on the other hand, are much stiffer, have higher values of Tg and Tm, and generally have much better properties. The overall synthesis and general structure of poly(ethylene terephthalate) [aka PET] is given below. You'll notice I said it's called an aromatic polyester, but that's only partially true. There's also an aliphatic component of the repeat unit that gives the polymer some flexibility. We'll contrast this mixed aromatic-aliphatic backbone with all-aromatic polyesters called "arylates" later. Turns out for melt processing, you really need this bit of added flexibility. Otherwise, what would use to make fibers for cotton-polyester blended shirts and pants?
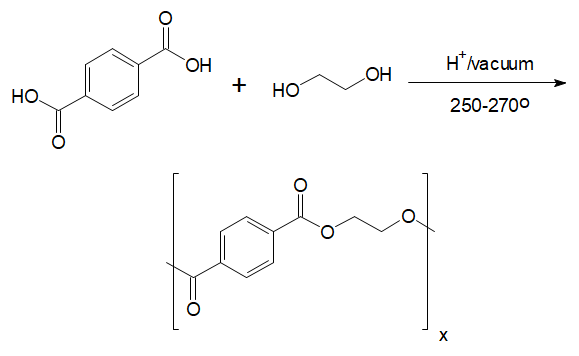
We'll first explore the general properties of both families, focusing on the ester group hybridization and contribution to backbone behavior. Then we'll move onto aliphatic polyesters, discuss a few common examples, delve into the mechanisms of polymer formation and including monomer syntheses details.
The aromatic polyesters are by far the more important family commercially, used as fibers, films and optical materials in a variety of applications. We'll compare various members of this group by examining both properties and specific examples of commercial products. Then we'll describe polymer synthetic methods, both academic and industrial, with details of monomer sytheses and mechanistic considerations.
Last we'll discuss recent developments in polyester syntheses and new monomer contributions to properties. For example, we'll examine synthesis of PBT macrocycles and their use in ring-opening polymerization with improved control of kinetics, polymer molecular weight and properties. Then we'll describe all-aromatic polyesters (also known as "arylates") which require more demanding synthetic methods but give enhanced physical properties.
Basic Ester Forming Reactions
Back to what we mean when we use the word “polymer.” Most polyesters (and lot of small molecule esters for that matter) are made by simple condensation of a carboxylic acid and an alcohol. That's the second from the top reaction below.
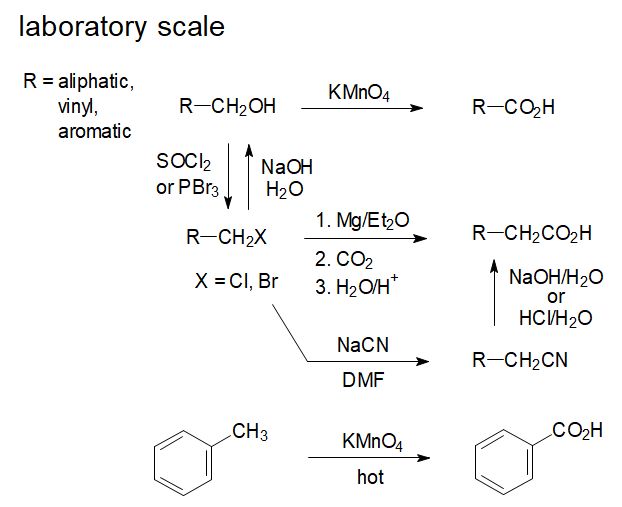
There are other ways to "trick" esters to form, however, and one of those are shown in the figure using the acid chloride. Why do we need to "trick" them into being? Good question. Answer is based on energetics: ester groups aren't really all that much more stable (if at all) than the combination of acid plus alcohol. Remember that free energy of reaction (ΔG) involves both enthalpy (ΔH) and entropy (ΔS). That is, ΔG = ΔH -TΔS, where "T" is temperature. We'll save in-depth discussion of this equation for latter but for now, the important thing to note is that the enthalpic term has to be greater than (overcome) the entropy loss term. That means the ester bond has to be lots more thermodynamically stable then an alcohol plus acid, and that's barely the case most times: hence, the need for tricks and such.
So how does this so-called sleight-of-hand work with the acid chloride? It's actually a two-part trick. First, you use a really high-energy species like the SOCl2 molecule to make the acid chloride. Because it's so high energy, it's a steep drop in energy to the acid chloride, even though THAT product of the reaction is actually much higher energy than the starting carboxylic acid. In short, we drive the carboxylic acid uphill energetically to the acid chloride. Reaction of that species with an alcohol is way downhill, so it goes fast and furious to give the ester without having to use acid catalysis and vacuum to force ester formation. Clear? If not, come back later for more on this fascinating example of why you need physical chemistry to do good organic chemistry (and vice versa).
And before we leave the figure above to its own entertainment, note that formation of both the amide group and the carboxylic salt are much more energetically favorable than ester formation. And that piece of information opens up vistas for making nylons (polyamides) from ester containing monomers, and for recycling polyesters back to monomers to be used over again...
Now for the reason you came to this section! To find out something about where polymers are actually used in everyday life. Well, we're going to kill two birds with one stone (another mysterious saying that we really don't want to pursue too deeply). Below you'll find the recycle symbols for six of the most common polymers in everyday use, plus the polymer that each represents. Also given are descriptions of several uses for each polymer.
To learn more than you ever wanted to know about the polymers in question, beyond what's on this list, just click on the name itself. Be advised, though, doing so will take you to our sister site, "The Macrogalleria," an exposition on many things polymer. And if you want even more examples of where polymers are used, visit Level 1 of the Macrogalleria where there are dozens of stores with polymer-containing things and stuff of all kinds. Just remember to follow your trail back here to continue with the last activity for this section: evaluation quizzes!
For now though, it's time for another (you guessed it!) quiz! Click here for your next evaluation quiz. It's a long one, covering more than what we've discussed in this course so far. But hey, that's a good way to learn other than by just reading a bunch of words on a page.
Good news! You've finished the introductory course on polymers- what they are, what they're made of, how they behave, and most important, where they're used. All good information that prepares you to dive into the details more deeply. The next course on Basic Polymer Synthesis will focus on the organic chemistry of making polymers. Key difference is this: a typical organic chemist is perfectly happy with a yield of 80 or 90%, but a polymer chemist has to have yields of over 99+%. "What?" you ask in amazement. "That can't be so, why, why, no organic reaction is that clean and complete."
Well, not to argue but in fact, it took us a long time to work how conditions and monomer purities that allow polymerizations to go to virtually 100% completion. Got your interest yet? Cruise on over to the synthesis course and find out more...
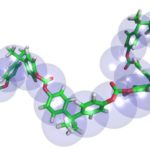