Analogies are dangerous creatures, never quite doing what you want them to do. Most analogies only represent part of the thing or concept you’re trying to illustrate. You try to give a visual picture of something complicated in the hopes that the viewer will be able to understand the analogy better than a detailed description of the actual thing. You try to relate something new to something the viewer already knows something about. This often fails. So in the analogies below, none of which are perfect, we’ll point out what is consistent with the real thing and what isn’t.
Train Analogy
One analogy often used is a railroad train which actually fits some of the properties of a polymer chain pretty well. Like many polymers, especially those made with a free radical initiator, there’s an “engine” at one end that gets the train started, and after the train is all put together, there’s a “caboose” which ends the train. At least, there used to be a caboose on a train- gone the way of all things to save money, I guess.
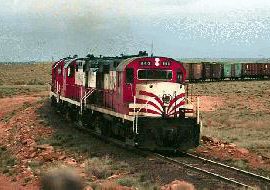
In a polymer chain, the “engine” is the initiator fragment that started the free radical polymerization (for that kind of polymerization). The “caboose” is the terminating group which stops the polymerization dead in its tracks (pun, get it?). That group results from a specific kind of reaction we’ll talk about later, the key point point being that the engine and caboose molecular fragments are different from each other and from all the “train cars” in between.
So just what are those train cars? Why, monomer units, of course! Notice I didn’t say monomers because during the polymerization process, the monomer undergoes a chemical reaction that changes it. In free radical vinyl polymerization, the monomer double bond (consisting of one sigma and one pi bond between the two vinyl carbons) is changed into a single sigma bond between the two carbons PLUS two new sigma bonds between each of the vinyl carbons and monomer units on either side (see the figure below). Think of how the coupling knuckle on a train car (one on each end) links up with the knuckles of the cars before and after it. It goes from “open” to “locked” when it links up with another car who’s knuckle also is transformed.
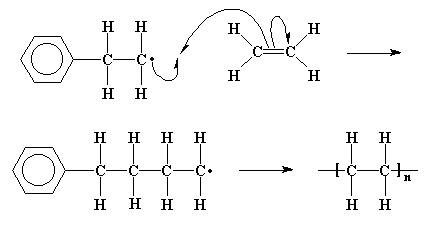
Now we see the real utility of the train analogy: a given train is made up of many cars that may all be the same or different, all linked together head-to-tail to give one long train. In polymer speak, many monomer units link together with chemical bonds to form one long polymer chain (a single macromolecule). Good enough so far.
Here’s where the analogy is misleading. A real train is huge, dwarfing even the tallest NBA basketball player, whereas a polymer chain is extremely small, dwarfed by even the shortest human on the planet. In fact, a polymer molecule can’t be seen by eye or even with really good optical microscopes. It takes a special kind of instrument, such as an electron microscope (hey, what?) to see an actual polymer molecule. Polymer molecules are even smaller than bacteria. We know bacteria exist because they cause infections, but many of us have a hard time believing how many bacteria there are all around us because we can’t see them with our own eyes. Did you know there are sometimes as many as 80 different kinds of bacteria living just on the skin of your elbow fold?
The other problem with the train analogy is that it doesn’t convey any sense of how a macromolecule actually behaves. A train moves only along the tracks it follows but a polymer chain can move in three dimensions plus it can change shape over time as it wiggles and squirms. Understanding that molecular behavior is important if you want to understand how the chemical composition of a polymer affects its physical properties. That understanding is especially important if you want to actually use the polymer for something. And that kind of understanding uses “structure-property relationships,” something we’ll talk about later in a different course (definition at the end of this chapter).
String Analogy
Ok, so a train doesn’t work all that well. What else can we use? How about string? A piece of string is certainly smaller than a train, thin and flexible, just like most macromolecules are thin and flexible. In fact, that flexibility leads to a very important property of strings: they get tangled up. Take a handful of strings of different lengths (just like the different lengths of polymer chains in a typical commercial polymer), mix them all up and try to pull one string out of the mess. If the strings are short, a few inches long, each one gets pulled out pretty easily. Make the strings longer, say a few yards in length, and it gets harder to disentangle a singe piece. Make the strings a hundred feet long and even the most patient person would take a long time removing just one from the tangled up mess.
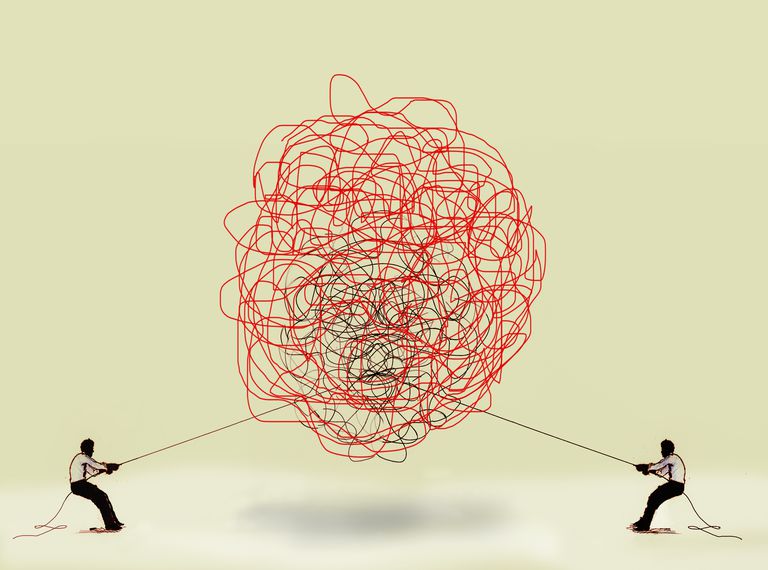
Now we’re getting a picture of how polymer chains behave: they tangle up with each other. Of course, even a piece of string is many, many times bigger than a macromolecule, but at least now we’re getting closer to the actual relative scale of a polymer. By that I mean that the length-to-width scale is closer for a piece of string a hundred feet long to what the length of a polymer chain is to its molecular width.
Here’s where this analogy breaks down: molecules move around a whole lot faster than we can move a piece of string. Sure, polymers tangle up, but because they’re wiggling and squirming and reptating (got you with that one!) so fast, they can disentangle faster than we can disentangle a ball of string. At least under the right conditions of temperature, that is. Freeze out molecular motion and polymer chains just sit there all tangled up. More on temperature later.
Our two analogies together give us different pictures of how polymer chains are made and how they behave. Neither works well by itself, but hopefully, you’re beginning to “see” polymers a little better now.
Spaghetti Analogy
I mentioned early that we’d talk about temperature effects on polymers. Here’s a brief introduction to one of the really unique properties of polymers, the glassy state and the glass transition. Take the “Basic Polymer” course to find out more, but for now, let’s just say that most polymers aren’t like most small molecules. Take a group of small molecules, cool them down and they become a solid. But, hey, what kind of solid is it? Turns out it’s usually crystalline. That means that it has a very regular placement of all the small molecules so that they all look the same throughout the solid. They’re all packed together neatly with the same orientation and the same distances to nearby molecules. Salt is a good example, or sugar. Look at their crystals and you can see regular shapes that result from the way all the individual molecules pack together. An analogy for such a crystalline array might be a box of pencils, all lined up and close together. Another might be dominoes packed into their container, or uncooked spaghetti in a bag or out. Get the picture?
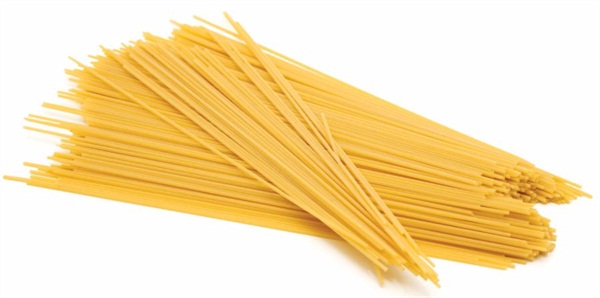
So here’s the weird part: polymers don’t generally behave this way. That is, they don’t pack all that well to form regular ordered structures that are uniform and consistent throughout. Oh, polymers can become “solid,” in a way, but not usually in a crystalline way.
Let's explore the spaghetti analogy a little further: buy spaghetti and it’s like a crystalline polymer with the chains (individual noodles) all lined up and close to each other. Take a look at the picture above and see if that makes you hungry. It should at least help you visualize crystalline polymer chains all lined up.
Now cook the spaghetti, especially if it's dinner time. You have to stir it as the water heats up, maybe add a spoonful of vegetable oil, to keep the strands from sticking together. Once it’s cooked, the hard noodles you bought are now soft and tasty, ready to mix with a meatball or two and some sauce (getting hungry yet?).
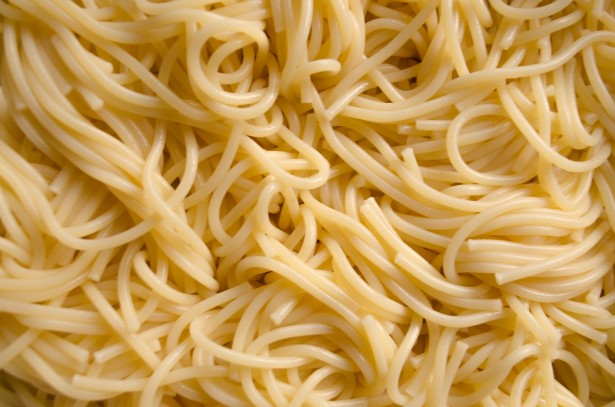
You finish dinner but have some noodles left in the serving bowl. Too tired to do dishes, you leave it till morning. When you try to pull the previously flexible noodles out of the bowl, they all come out together, stiff and tangled up in one big solid blob. Key point: they dried out and got hard again, but don’t go back into the close-packed way they were in the package you bought. Yes, they’re solid but definitely not “crystalline,”.
Polymer chains do the same kind of thing at the molecular level, going from solid (that’s not crystalline) to a soft and flexible mass that’s all tangled up. Usually this “glass” transition happens by heating but it could involve solvent just like the hot water used for spaghetti. Here’s the key point: cool the polymers down and they become solid again, but just like the dried spaghetti you fed your dog, that solid form isn’t crystalline. It’s just a mess of tangled up, unmoving polymer chains.
The Snake Pit
Ok, now let’s get a real visceral feel for polymers. Warning: those of you who like snakes or have one as a pet may find the following disturbing. Caveat: not a single snake was harmed in the making of this analogy.
So what’s this “snake pit” polymer analogy all about? Let’s talk about why polymers with no order to them are hard and brittle below a certain temperature and soft and pliable above it. It can help to think of a polymer in the amorphous state as a big ball of slithering snakes. Each snake is a polymer chain (but a short one). Now as you may remember, snakes are cold blooded animals, so all their body heat has to come from their surroundings. When it’s warm, the snakes are happy, and can go on about their business of slithering and sliding with no trouble at all. They will move all about randomly, over and around each other, as they slither hither and thither, just having a great time, or as good a time as snakes ever have.
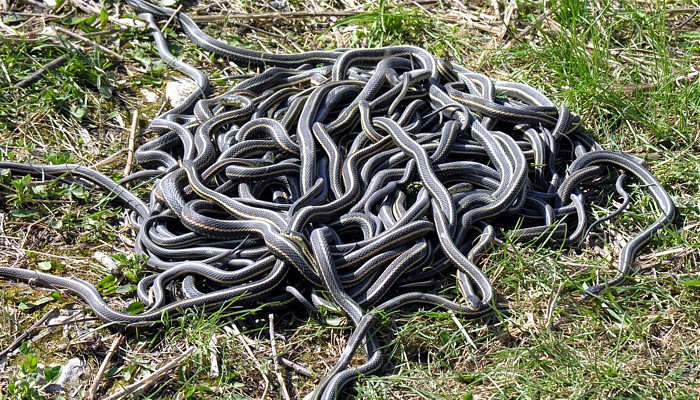
But when it gets cold, snakes don’t move very much. They slow down without any heat, and tend to just sit still. Now in our ball of snakes, they’re still all wrapped around, over, and under each other, but as far as motion is concerned, it just doesn’t happen.
Now imagine trying to drive a bike through this room full of snakes. If it’s warm, and the snakes are moving fast, they can quickly slither out of your way. You and your bike move through the snakes, causing a minimal amount of snake damage. But if it’s cold, one of two things will happen to the motionless snakes. Either (A) the snakes will be stronger than the bike, you won’t get through and the snakes will stay put; or (B) the bike will be stronger than the snakes, and they’ll get squashed, still not moving anywhere as they become pulped.
Polymers are the same way. When the temperature is warm, the polymer chains can move around easily. So when you take a piece of the polymer and bend it, the molecules, being in motion already, have no trouble moving into new positions to relieve the stress placed on them. The piece of polymer simply bends. But if you try to bend a sample of a polymer below its Tg (or glass transition), the polymer chains won’t be able to move into new positions to relieve the stress. So just like a room full of cold snakes, one of two things will happen. Either (A) the chains are strong enough to resist the force you apply, and the sample won’t bend; or (B) the force you apply will be too much for the motionless polymer chains to resist, and the polymer sample will break or shatter in your hands.
This change in mobility with temperature happens because of the phenomenon we call "heat" which is really a form of kinetic energy. That is, heat equals the energy of objects in motion. It actually involves random motion of molecules, whether they are polymer molecules or small molecules. Things are "hot" when their molecules have lots of kinetic energy and move around fast. Things are "cold" when their molecules lack kinetic energy and move around slowly, or not at all.
Summing Up
Ok, using the four analogies above, we now have a picture of both what polymers chains look like and how they behave. One causes the other. The extreme length of a polymer chain relative to its width results in molecular behavior that causes physical or macroscopic behavior, the kind we can see and feel with our own hands. The next lesson will explore the basic properties of polymers in more detail. For now, though, it’s time for another (you guessed it!) quiz! You may not (probably don't) know the answer to some of the questions, but that's ok; we'll get to them later. For now, just take the basic version here or the more advanced version here for your next general evaluation quiz.
Now that you have a sense of the unique properties and behavior of polymers, it's time to look closer at their molecular compositions and how those impact macroscopic properties: Polymer Compositions.
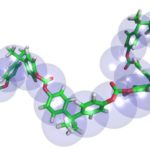